To the ends of the earth and beyond – the search for 'impossible' quasicrystals
Hello all, Helen here! After a mad few weeks of traveling, I thought today I would lean on some 'existing material'. Here's an article on quasicrystals that I wrote back in 2012 for The Conversation.
In 2010, an international team of scientists reported that a sample of natural "quasicrystal" found in a Russian mountain range had formed 4.5 billion years ago and arrived on Earth as a meteorite. The discovery was the latest episode in a decades-long struggle to understand the nature and origin of quasicrystals – a mineral that was first thought "impossible".
So what exactly are quasicrystals? What makes them "impossible"? And how did the "impossible" finally become possible?
The story starts with a bit of basic materials science.
In solids, atoms can only arrange themselves in a few restricted ways. There are materials with no order (called amorphous solids), such as glasses. Then there are crystalline materials – such as diamond or amethyst – which have a collection of only a few atoms repeated again and again.
Within the crystalline category, atoms are very restricted in the symmetry of the arrangements they take up: only two-, three-, four- and six-fold symmetries are possible. (Put simply, an n-fold symmetry refers to a shape that needs to be rotated n times before it returns to its original position. A triangle has a three-fold symmetry because it needs to be rotated three times before returning to its original position.) You can test these symmetries for yourself to a point. If you draw a triangle on a piece of paper you can stack triangles around it to build up a pattern without leaving gaps.
You can also do this with squares, rectangles and regular hexagons, but you won't be able to do it with a pentagon without gaps (nor for any shape with an odd number of sides, beyond triangles).
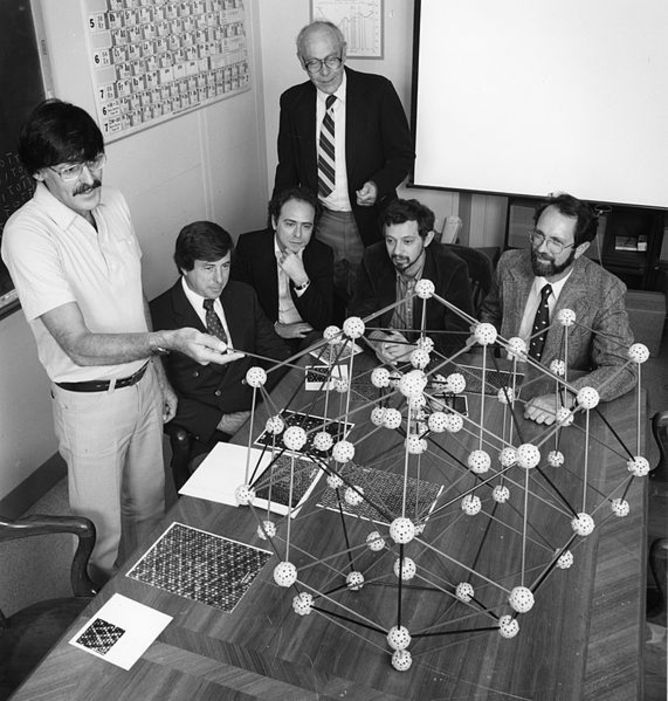
In 1984, Israeli materials scientist Dan Schechtman was on sabbatical in the US when he analysed a rapidly cooled metal alloy. His data showed the atoms in this alloy were arranged to have five-fold symmetry.
Despite being puzzled by the impossibility of what stared back at him, Dan Shechtman had the fortitude to publish his results and 17 years later, in 2011, he was awarded the Nobel Prize in Chemistry for his discovery of what would later be called quasicrystals.
But the journey from discovery to Nobel Prize was far from smooth. Shechtman encountered much opposition to his observations, most notably from two-time Nobel Prize winner Linus Pauling who suggested: "Danny Shechtman is talking nonsense. There is no such thing as quasicrystals, only quasi-scientists."
It would seem that Schechtman received his Nobel Prize as much for his humility and courage in sticking by his findings, as he did for making those findings in the first place. There's also the fact that what he discovered was, truly, very beautiful.
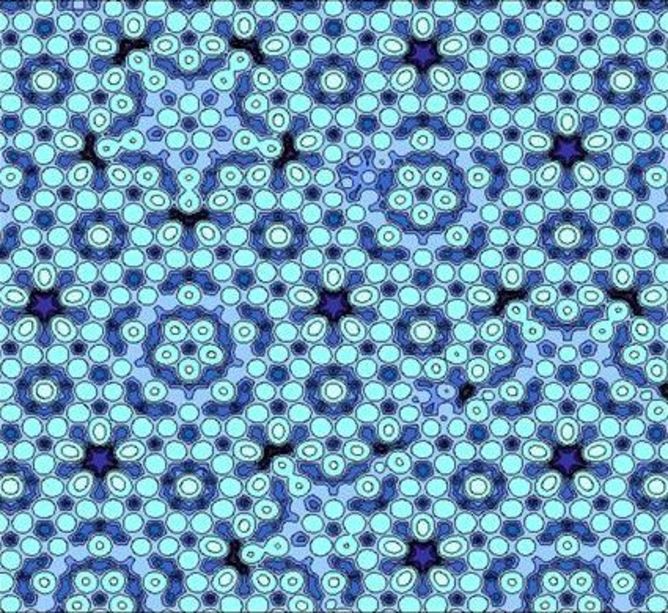
Elsewhere in the US, another group, lead by Princeton physicist Paul Steinhardt, had already theorised that these quasicrystals were mathematically possible and were hot on the hunt for them in nature.
Steinhardt (along with his colleague Dov Levine) was the one who coined the term "quasicrystals" to describe the 3D models he got from stacking boxes akin to the Penrose tilings. Penrose tilings work around the restrictions of symmetry by stacking two different shapes in two dimensions. Steinhardt and Levine extended this idea into the third dimension.
By stacking boxes, Steinhardt was able to create apparently "impossible" symmetries and build theoretical crystal structures that didn't quite repeat themselves. These quasicrystals beautifully reproduced Shechtman's results, but the big question remained: could one of these strange arrangements be found in a natural mineral crystal?
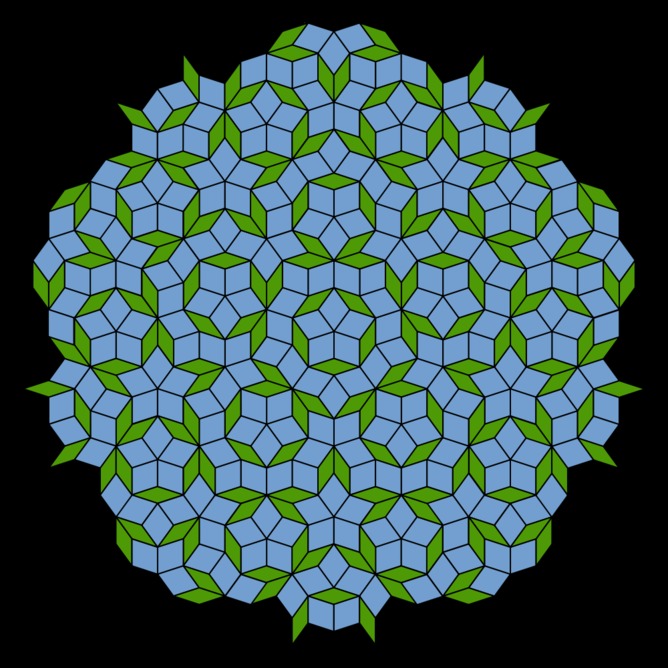
Since the initial work done by Shechtman in the 1980s, about 100 quasicrystals have been created in the lab – all metal alloys. In searching for quasicrystals in nature, Steinhardt and his team decided to hone in on minerals with similar metal composition to the alloys; a mixture of aluminium, copper and iron in particular.
In their search they came across the little known and rare mineral Khatyrkite, made up of this metal mixture, originating from the Kamchatka region of Russia. The thought was that any rock with Khatyrkite in it may also have a small amount of an unknown mineral near it that would have the right composition to make a quasicrystal.
Many will only know of the Kamchatka peninsula because of its inclusion in the board game Risk. As a region it truly is at the end of the Earth. So, before travelling there, Steinhardt's group put a call out to all the major mineral collections to find a rock with some Khatyrkite.
Eventually, one was located in a museum in Florence. To the researchers' delight, detailed analysis of the Italian rock revealed the presence of a new mineral with the five-fold symmetry – the first natural quasicrystal, later named icosahedrite.
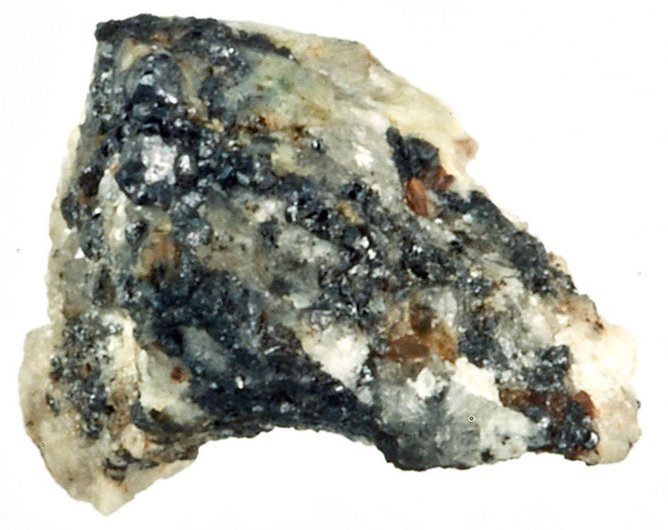
Paul Steinhardt.
The final twist in this tale came about from the smallest of clues. Closer examination of a grain of sand within the Kamchatka rock revealed it wasn't quite the type of silica sand you would find on the beach.
This grain of sand was found to be stishovite (or "shocked quartz"), an arrangement of silica atoms that can only come about under massive pressures – such as the pressures caused by the impact of a large lump of rock falling from a great height.
Published in the Proceedings of the National Academy of Sciences in January, Steinhardt and colleagues presented detailed evidence from the oxygen chemistry, showing the rock containing isocohedrite is more similar in composition to meteorites than it is to other terrestrial equivalents.
It would seem that the first natural quasicrystal was actually made in space and probably right at the beginning of our solar system, making it one of the first minerals to be formed.
Quasicrystals started as an interesting mathematical exercise, before angering the most eminent of scientists and then, finally, changing the way we define minerals. The search for quasicrystals was pushed to the ends of Earth and then into space.
You really do have to wonder where these impossible crystals will take us next.
Further reading:
Helen Maynard-Casely does not work for, consult to, own shares in or receive funding from any company or organisation that would benefit from this article, and has no relevant affiliations.
This article was originally published on The Conversation.
Read the original article.